by Shane L. Larson
In the world of artistic painting, connoisseurs have a word: pentimento. It is the revelation of something the artist hid from us. There are many reasons why changes to a composition may come to light. Sometimes it is because as paint ages, it becomes more translucent, revealing a previous facial expression or position of a hand. Sometimes, close and careful study reveals that a slight alteration was made to disguise a mistake or a shift in ideas about the composition. And still sometimes technology can be used to see through the painting to what lies beneath — the artist’s original sketch or painting that was altered in the final production
The word pentimento is an Italian word, meaning “repentance.” Its use in the context of art is an implication that the artist has been caught red-handed, changing their mind about a particular composition! The idea of repentance and being caught red-handed carries a certain amount of emotional baggage in our culture; I suspect it is ingrained in us at an early age, when our parents catch us doing something we’d rather them not know — like stealing cookies from the cookie jar, or seeing what we look like if we cut our eyelashes off, or getting caught reading Scientific American under the covers with a flashlight (I just made all of those things up — my parents never caught me doing any of those!).
But science is different. Part of the game is about being wrong and getting caught. There is no shame in changing your mind, no repentance for previous incorrect speculations about the nature of the Cosmos. You make up cool ideas, that you present to the world not as art, but as proposed mathematical explanations for how the Cosmos works. Any crazy idea is fair game, with one requirement: you have to also suggest a way for us to do an experiment to test if your crazy idea is right! If it’s right, we go think of new experiments; if it is wrong, then we look at your crazy idea and figure out which crazy bits of it aren’t quite right. We make some changes, turning it into a new crazy idea, and then go conduct another test.
Science is always these two parts — the first part, describing the world, is called “theory”; the second part, testing your ideas, is called “experiment.”
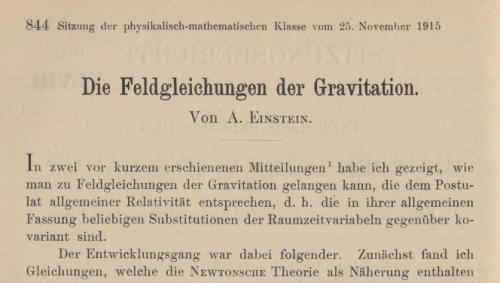
The leading header of the paper where Einstein introduced general relativity, his writeup of the presentation he made to the Prussian Academy of Sciences in November, 1915.
Albert Einstein introduced General Relativity to the world in a presentation to the Prussian Academy of Sciences in November of 1915; his written summary of that presentation may be read (in German) online: Die Feldgleichungen der Gravitation (“The field equations of gravitation”). If you look at that paper, you will see it looks a lot like your introductory physics textbook looked — lots of mathematical symbols and equations. What does it all mean? This is the “theory” side of gravity, where we imagine the Laws of Nature that describe gravity — in this case, the idea that gravity can be explained as the curvature of spacetime.
General relativity was a tremendous step forward in our understanding of gravity — it was consistent with special relativity and extended our understanding into physical regimes that Newtonian gravity could not address. But at the same time, especially early on, very little was actually known about GR. It was mathematically difficult to work with (in the lingo of physics, it is “non-linear”), and in 1915 there were no known astrophysical systems that absolutely required general relativity to describe them. Einstein knew it was fine to write down new and possibly crazy ideas about the Universe, but the real task was to decide if there were ways to test those ideas. Were there some observations that could be made and compared against the predictions of general relativity? Observations that confirmed the predictions of general relativity would demonstrate its viability as a description of gravity. There have been many tests of general relativity discovered over the course of the past 100 years, but Einstein himself set the stage for testing his ideas by proposing three immediate tests that scientists could put their efforts into.
The first test was one that Einstein used to convince himself that general relativity was going in the right direction. In 1859 Urbain Le Verrier had noticed something odd about Mercury’s orbit. Like all planets, Mercury has an elliptical orbit — it is a slightly squashed circle, closer to the Sun on one end than on the other. The point where the orbit comes closest to the sun is called perihelion, and it lies in a particular direction. Over time, the direction to perihelion changes — the orbit of Mercury pivots slowly, in a dance that astronomers call precession.

The point of closest approach to the Sun is called perihelion, and occurs in a certain direction (green dashed lines). Over time, an orbit pivots slowly — it precesses — changing the direction to perihelion.
Some precession is expected, because the Sun is not a perfect sphere (it is actually a bit squashed, fatter at the equator because it is spinning), but Le Verrier had looked at 150 years of observations of Mercury’s orbit and discovered the perihelion was shifting by an anomalously large amount — 43 arcseconds every century! That is to say, the angle of Mercury’s orbit was pivoting by an extra angle over the course of 100 years, equivalent to 43 arcseconds. How big is 43 arcseconds? Take a quarter and put it 382 feet away (a bit longer than a standard US football field) — 43 arcseconds is the angle between pointing from one side of the quarter to the other side of the quarter. It is a VERY small angle! But astronomers had detected this small change in Mercury’s behaviour through diligent and careful observations of the Cosmos.

43 arcseconds is about the apparent visual size of a US quarter when viewed from a distance of 382 feet (116.4 meters), slightly farther than the length of a regulation NFL football field.
When he was developing his new way of thinking about gravity, Einstein realized that the anomalous precession might be described by general relativity. He calculated that general relativity predicts an extra 43 arcseconds in perihelion precession for Mercury, the exact amount observed by astronomers. This not only resolved a 50 year old mystery in astronomy, but firmly convinced Einstein he was on the right track.
The second test is arguably one of the most famous tests in the history of gravity, and its success catapulted Einstein into the public eye, making him a world-wide celebrity. One of the central features of general relativity is that everything experiences gravity — everything “falls.” This is certainly true for things like rocks and slurpees, but Einstein also realized it should apply to light. Light, like all freely moving objects, wants to travel in a straight line, and generally it does so. This is one of the features that makes it such a useful messenger in astronomy: if you receive some light on Earth, and look back along the direction the light came from, you should be looking at the object that generated the light!
![[A] When viewed alone in the sky, two stars (yellow and red) have a well defined separation, defined by the direction you have to point to look directly at them. [B] They appear separated, and that amount can be measured. [C] During a total solar eclipse, light from the yellow star passes near the Sun and is bent. Looking back along the line of sight, the yellow star appears to be closer to the red star than it was when the Sun was not in the way. [D] The deflection of starlight is the amount the position of the yellow star appears to move on the sky.](https://writescience.files.wordpress.com/2015/02/deflectionstarlight2.png?w=500&h=647)
[A] When viewed alone in the sky, two stars (yellow and red) have a well defined separation, defined by the direction you have to point to look directly at them. [B] They appear separated, and that amount can be measured. [C] During a total solar eclipse, light from the yellow star passes near the Sun and is bent. Looking back along the line of sight, the yellow star appears to be closer to the red star than it was when the Sun was not in the way. [D] The deflection of starlight is the amount the position of the yellow star appears to move on the sky.

One of Eddington’s images recorded during the 1919 Eclipse. The small horizontal hash lines mark the stars that would be measured.
So how can you measure the bending of light predicted by general relativity? The trick with light is if you want to see the deflection, it has to pass through a strong gravitational field. Einstein suggested you could look for the deflection of starlight during a total solar eclipse. The gravity of the Sun is strong enough to bend the path of light by a measurable amount; during an eclipse when the Moon blocks most of the light from the Sun, the stars near the edge of the Sun’s disk should be visible.
The first realization of this test was organized in the United Kingdom by the Astronomer Royal, Frank Watson Dyson, and Arthur Stanley Eddington. Eddington led an expedition to the island of Príncipe, off the west coast of Africa, to observe the total solar eclipse on 29 May 1919. Eddington imaged several stars around the eclipse, and confirmed general relativity’s predictions. These measurements are difficult to make, and their accuracy has often been debated, but the experiment has been repeated during many eclipses since then, continuing to confirm the predictions of general relativity.

The leading header of the paper summarizing Eddington’s measurements to confirm the deflection of starlight.
The last proposed experiment is called the gravitational redshift. Think about tossing a rock up in the air. What happens? When the rock leaves your hand, it has some initial amount of energy that physicists call “kinetic energy” — energy associated with motion. As it climbs, it slows down. It looses kinetic energy, expending it to fight upward against gravity. Einstein argued based on the Equivalence Principle that a photon must also expend energy to climb upward against a gravitational field.

Photons travelling upward in a gravitational field lose energy, becoming redder. Photons travelling down gain energy, becoming bluer.
But photons — all photons — propagate at the speed of light! The notion of “kinetic energy” as it applies to objects like rocks is hard to extend to photons. But the Equivalence Principle demands that a photon climbing up through a gravitational field must give up energy. How? It can change its color. Photon energy is directly related to its color — blue light is more energetic than green light which is more energetic than red light. A photon can give up energy as it climbs upward against gravity by changing its color, shifting from bluer light toward redder light.
Measuring the change in color of light is easy to do, but notoriously difficult to attribute to general relativity because all kinds of things change the color of light! But in 1959, Robert Pound and Glen Rebka successfully measured the gravitational redshift at the Jefferson Laboratory at Harvard.
Pound and Rebka’s triumphant measurement concluded a more than 40 year effort to complete the three classical tests proposed by Einstein in 1915. Since those early days, many other tests of general relativity have been suggested, and measured. To date, no experiment has uncovered any chinks or holes in the theory. If there had been, then general relativity would have been relegated to the trash bin of Cool Ideas that Failed, and we would have moved onward to look for a new understanding of gravity. Instead we find ourselves in that happy frame of mind where we use general relativity to describe the Cosmos with swagger and aplomb. There may yet be another revolution in our understanding of gravity, but if there is, I am confident that it will have to successfully include both general relativity and Newtonian gravity as parts of its core infrastructure.
In the end, there is a bit of pentimento in the game of science, but it is not on our part — it is Nature’s. General relativity is the latest in a series of tools that we have developed and used to peer closely at Nature. Slowly — ever so slowly — we are seeing through the paint Nature has clothed herself in. The secrets of the Cosmos are becoming slowly transparent, revealing the clockwork wonder of the Universe that hides beneath.
—————————————
This post is part of an ongoing series written for the General Relativity Centennial, celebrating 100 years of gravity (1915-2015). You can find the first post in the series, with links to the successive posts in this series here: http://wp.me/p19G0g-ru.
Pingback: BepiColombo blasts off in pursuit of Einstein | astrobites
Pingback: BepiColombo despega en busca de Einstein | Astrobites en español
Pingback: BepiColombo takes off in search of Einstein - Be Viral
Pingback: BepiColombo despega en busca de Einstein - Tecnologias Online